We have previously looked at the various properties of water which have an effect on aquatic fauna, some of them a bit out of the ordinary, such as surface tension. However, one of the most important
Contributed by
As pure water is only a liquid between 0ºC and 100ºC, it is not surprising that life, at least as we know it, which depends on water, is to be found on earth where this condition obtains, though most life on Earth lives at temperatures of less than about 50ºC.
Yet, the so-called hyperthermophiles can live in extremely hot environments i.e. hotter than about 60ºC, with an optimal temperature above 80ºC. The most heat-tolerant hyperthermophile is the recently discovered Strain 121, which can exist at temperatures of up to 121ºC. The toughest hyperthermophiles live on the superheated walls of hydrothermal vents, and require temperatures of at least 90ºC to enable them to survive.
The present temperature of the oceans
The oceans store vast amounts of energy in the form of heat, which has the sun as its primary source. Infrared radiation from the sun plays an important role in that ultimately all the forms of energy in the oceans, such as the long-term circulation and the evaporation at the surface of the sea, depend on input from the sun. There are only two sources of energy that do not depend on solar heating. These are tidal energy derived from the gravitational pull of the sun, moon and—to a small extent—the planets; and geothermal heating through the seafloor from the molten core of the Earth.
The net heat input to oceanic heating is given by the direct solar input and geothermal heating. These are estimated at 150 watts/m2 and 0.01 watt/m2 respectively. So, it is obvious that the geothermal heating is negligible compared with the solar heating. The net heat loss is given by black body radiation (50 watt/m2), the conductive heat loss to the atmosphere (10 watts/m2), and the loss due to evaporation (90 watts/m2).
The amount of sunlight arriving at the sea surface varies, of course, according to the time of day, season, and weather.
The temperature of the oceans is thus given by a dynamic balance between incoming heat and outgoing heat. There are naturally great differences, though, in the temperature of the water between, say, equatorial waters and polar waters, at least for the surface waters.
These surface water temperatures range from some 40ºC in shallow tropical lagoons to –1.9ºC (the typical freezing point of sea water) in polar regions. Apart from the Mediterranean and Red Seas, any warm water in the open ocean is restricted to an upper mixed surface layer of about 100 – 200 meters depth.
However, whatever the surface temperatures are, the temperature falls to about 5ºC at about 1000 m depth and thereafter declines slowly to between about 0ºC and 3ºC at greater depths. Even below the hottest tropical regions, the water at a depth of 2000 to 3000 m hardly ever rises above 4ºC. There are, of course, some few exceptions to this, for example the water close to hydrothermal vents where the water can emerge at temperatures of up to 400ºC, although it rapidly cools to the surrounding water temperature of 3 – 4ºC. (See X-RAY MAG issue 5, 2005)
Increasing ocean temperatures
There are various opinions about the magnitude of the temperature changes occurring due to global warming. However, the Intergovernmental Panel on Climate Change, based on a consensus of many hundreds of scientists from many countries, state that the global average surface temperature has increased over the 20th century by about 0.6ºC ± 0.2ºC. It should be noted, though, that this warming has not been globally uniform. The most recent warming has been greatest between the latitudes 40ºN and 70ºN, while the North Atlantic Ocean, for example, has cooled in recent decades.
These temperature changes are, however, still mostly confined to the upper water levels. Work by the Scripps Institution of Oceanography has modelled the time variation of heat content for the various ocean basins. In both the simulations and observations, the heat content in most of the oceans increased only slowly with depth, consistent with a diffusion process. The water had been warmed below about 1000 meters only in the north and south Atlantic, reflecting strong vertical convection there.
The mean daily variation in surface temperature in the open ocean is, however, very small, being generally less than 0.3ºC. Below ten meters depth, there are practically no variations in temperature. Unlike for creatures living in inter-tidal waters, which can be subjected to great differences between day and night-time temperatures, plankton and fish are unaffected by temperature changes over 24-hour periods.
Not only are there vertical changes in temperature, there are also the oceanic currents, which circulate the warm waters and the cold waters. Whilst most marine life has evolved as a function of the environments brought about by these regular currents, irregular currents such as El Niño can cause biological and physical functioning to change quickly over both small and large areas. It can disrupt the whole marine food chain and can be catastrophic for some species such as the Peruvian anchovy.
Although there might be some disagreement about the magnitude of the increase in oceanic temperatures, one thing is certain: even small changes in sea surface temperature can have drastic effects. There will be changes in oceanic circulation patterns, the polar ice will melt (it is already starting to doing so) giving a rising sea level, and giving fresh cold water which, being more dense, tends to sink.
These variations in temperature and salinity will control vertical ocean currents. Water temperature also partially determines the concentration of dissolved gases, such as oxygen and carbon dioxide, in sea water. These gases are fundamentally linked to biological processes. All these factors will effect life in the oceans.
Effect of increasing temperature on biological processes
Biological processes, like all other chemical processes, are rate-dependent on temperature, and biological molecules are generally very sensitive to increased temperature levels. The rate of reaction increases with increasing temperature up to a certain point, where either the reaction reaches an equilibrium or the reactants/products decompose. An exaggerated example, perhaps, but just think of a boiled egg where the proteins become irreversibly changed during the four minutes or so at 100ºC.
To counter the effect of high temperatures in nature, the thermophiles, for example, mentioned above, have had to evolve special proteins, as DNA will normally be denatured above 60ºC. Denatured means that the DNA helix strands separate. When double stranded DNA is heated up to between 60 to 80ºC, the two strands unbind into single strands. They will recombine if allowed to cool slowly. Such organisms will probably be totally unaffected by any possible increase in the Earth’s temperature.
The temperature dependence of the rate of chemical reactions
A rule of thumb is that for most simple chemical reactions the rate approximately doubles for every 10ºC increase in temperature. Thus a reaction at 35ºC will go about twice as fast as at 25ºC. However, biological reactions are far from being simple from the chemical-kinetics point of view. The rate of metabolism, as measured by oxygen consumption, of all poikilothermic organisms is greatly increased with rise of temperature. According to van’t Hoff’s rule, the increase is two to three times for each 10ºC rise in temperature within favourable limits. This can be of great consequence for life on earth in the years to come.
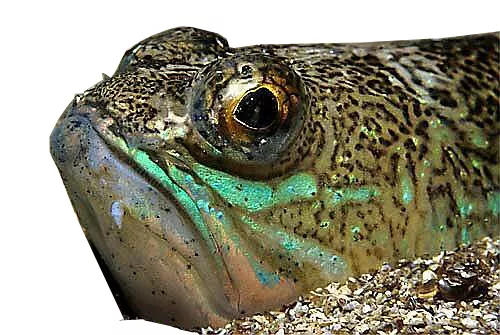
Effect of temperature on marine animals
Marine animals, like their terrestrial counterparts, can be divided into four main types with regard to their thermal behaviour.
Firstly, there are the animals whose body temperature varies with the temperature of the surroundings. They are the poikilothermic animals, the so-called cold-blooded animals, (from Greek, poikilos, various), these being the invertebrates and fish. It is well known that different poikilothermic animals differ greatly in their ability to tolerate high temperatures although the time of exposure is also very relevant.
This is in part due to the effect of temperature on nerve velocities in peripheral nerves, which can have sharp upper and lower limits. For example, in some Antarctic poikilotherms heat-block was found to occur at around 31ºC. Poikilothermic animals generally have more complex metabolisms than the homoiotherms (warm-blooded creatures), having four to ten enzyme systems that operate at different temperatures.
In general, poikilotherms do not use their metabolisms to heat or cool themselves although the swimming muscles of the Tuna fish are warmed by a heat exchanger, with a network of fine veins, the rete mirabile, providing a thermal barrier against loss of heat. One obvious means of temperature control for poikilotherms such as fish is to change depth in the water column to find a suitable temperature.
Secondly, there are the stenothermic species (from Greek, stenos, narrow) which are restricted to narrow temperature limits. These include the reef-building corals, for example, which require a minimum temperature of 20ºC and a maximum temperature of not much more than 30ºC.
Species, which are cold–stenothermic, have a wide geographical range, being found in the shallow waters of the Arctic as well as at depths of 2000 – 3000 m where the temperatures are about 4ºC. An example is the bald rock cod, Pagothenia borchgrevinki, a common fish which lives under sea ice at temperatures of –0.5ºC to –1.8ºC , and which dies at temperatures over 6ºC (this is the lowest known temperature to kill an animal). However, in the long term this may not be true, see below.
Thirdly, there are the animals that can exist in environments with a wide temperature range; they are known as eurythermic (from Greek, eurus, wide). These species tend to have wide distributional ranges, or they live in regions of considerable temperature fluctuations, such as temperate inter-tidal zones. Such animals are, for example, the periwinkle (Littorina litorea), the common mussel (Mytilus edulis) and the common cockle (Cardium edule).
Finally, there are the aquatic mammals that are homoiothermic (from Greek, homos, same) i.e. they maintain a constant body temperature, and are usually referred to as warm-blooded. These are the cetacea, discussed in a previous issue, that have sophisticated mechanisms for maintaining body core temperatures, which are close to that of humans, 37ºC. For example, the Humpback whale has a body temperature of 36ºC, with the average body temperature of Cetaceans being ca 35.5ºC, which is low for a mammal. In comparison, the elephant seal has a body temperature of 36.7ºC and the Weddell seal 37ºC.
Control of temperature in aquatic mammals
In terrestrial mammals, temperature is controlled by a number of mechanisms. The central organ for temperature control is situated in the hypothalamus, which contains the control mechanisms as well as the key temperature sensors. The body core temperature is detected by these sensors, which change their rate of nerve impulse generation according to the temperature. At the normal body temperature, these cells generate pulses at a certain rate which indicates the pulse set point of the system.
If, for example, the core body temperature falls below 37ºC, then the nerve impulse rate slows down, and a message is sent to the hypothalamus. The body will then initiate a number of positive responses to conserve heat in the body and to increase heat production, and with immediate cessation of sweating.
Flow of heat to the skin will be reduced by vasoconstriction of the capillaries, shivering will be induced to increase heat in the muscles, and there will be secretion of the hormones norepinephrine, epinephrine, and thyroxine to increase heat production. There may also be ‘goosepimpling’ of the skin, which raises the hairs on the body. This is a residual effect from our evolutionary ancestors who were covered in hair or fur, and erected the hairs of the fur to increase insulation.
If the body temperature is too high, it can be cooled by radiation, conduction, convection, and evaporation of sweat. The first three factors are not under control by the body; they are purely physical effects that depend only on the actual temperature of the body and the ambient surroundings. Naturally, we humans can increase their effect by removing clothing, to improve radiation, or fanning our bodies to increase conduction and convection. However, the body itself can actively induce sweating, so that if the skin temperature increases above 37ºC, sweating will begin almost immediately.
Like in their terrestrial relatives, the aquatic mammals, too, have a very sophisticated temperature control system. Although very similar, it is obvious that some of the mechanisms used by the human body cannot be used by the aquatic mammals. Sweating, for example has no function in controlling the temperature of these creatures.
Whales require a thick layer of blubber to maintain their body temperature in water because heat is transmitted much more easily by water than by air. Up to 45 percent of the body weight of a whale can be made of blubber, which serves not only as an insulator but also as an energy reserve.
Ocean water is relatively cold in contrast to body temperature, but a fast swimming whale does produce a lot of heat, which has to be removed. This is carried out by means of blood vessels leading to an extensive network of capillaries in the flippers, tail flukes and dorsal fin.
Concurrent circulation, on the other hand, minimises heat lost from the arteries to the surroundings. In some ways, temperature control may be easier for the cetacea, for it is easy to get rid of heat in cold water—if the water is cool enough.
Adverse effects of increasing oceanic temperatures
It has been discovered that global warming caused marine mass extinction at the end of the Permian period 251 million years ago. It is estimated that 95 percent of marine species were killed off. This mass extinction, although the worst, was but one of five that have occurred over the past 550 million years. The temperatures are estimated to have been about 6ºC higher than today. The most recent event was the Cretaceous-Tertiary, which occurred 65 million years ago, when temperatures were about 4ºC higher than today.
The mass extinction 251 million years ago appears to have happened because of the slow decline and death of deep sea creatures, which were the first to go, followed by the shallow water inhabitants. It indicates that something was arising from the deep oceans which possibly was the very toxic hydrogen sulphide produced by deep-ocean anaerobic bacteria i.e. those that don’t use oxygen in their metabolism.
These bacteria were favoured by the fact that the warming of the surface water had decreased the ability of the oceans to absorb oxygen. However, whatever mechanism it was that caused the mass extinction, it was probably tied to the ocean circulation processes. The emission of hydrogen sulphide from the oceans could also account for the great demise, 65 percent of the terrestrial species, which occurred at the same time.
Will a rise in temperature lead to a new mass extinction? Whether or not a mass extinction is soon to occur, it is believed by some climatologists that a rise of 1.5ºC to 2.5ºC is likely to take place before the middle of the century. If this is true, then between 20 percent and 30 percent of animal and plant species could die.
Sea water temperature affects faunal distribution and, as we have seen, water temperatures can vary both horizontally in the surface and vertically down into the depths. So, these temperature changes have a great significance for the faunal—and floral—distributions. Faunal distribution depends to a large extent on a given organism’s ability to adjust to the ambient conditions. If they cannot adjust, they must either move or die.
For example, the eelpout, Zoarces viviparous, is near the top of its local food chain in the shallow waters of the southern North Sea. Research carried out at the Alfred Wegener Institute in Bremerhaven, Germany, appears to show that the fish face an oxygen constraint because as the water warms up, it contains less dissolved oxygen. With less oxygen available, the eelpout gives birth to fewer young, so their population declines.
This also appears to be the case with cod in the North Sea, which are finding it hard to maintain high reproducibility. A permanent increase in water temperature will probably not mean that these fish will become extinct, but that they will move from their ancient homes to new, cooler aquatic pastures to the north.
The invasion of jellyfish
A typical example of what happens ecologically when the sea temperature changes even slightly is given by the mauve stinger jellyfish. Recently, millions of these creatures drifted into a salmon farm in the Irish Sea, killing more than 100,000 fish. Several more swarms of these jellyfish, a Mediterranean species, have been observed around Britain, even as far north as the Shetland Islands.
Apparently, warmer sea waters in the Mediterranean is boosting the jellyfish numbers by increasing winter survival and lengthening the breeding season. The increasing water temperatures then allows them to move north. Generally speaking, most jellyfish are positively affected by global warming, not only by the increasing water temperatures, but by the fact that the increased concentration of carbon dioxide affects creatures with acid-soluble shells which compete with jellyfish.
Because they have positive control over their body temperatures, the homoiothermic animals will probably be the least affected by temperature increases, at least initially. Those best suited to survive will therefore probably be the aquatic mammals which, like humans, have a large measure of control over their body temperatures thus making them, to a large degree, independent of increasing water temperatures.
The first type of aquatic animal to be really effected are those who are restricted to narrow temperature limits i.e. the stenothermic animals such as the corals. There is already evidence that this is occurring with the bleaching of corals.
The eurythermic animals, which have evolved to survive in environments with a wide temperature range, will probably have little difficulty in adapting to increased temperatures.
The greatest effect of changing temperatures will obviously be on those creatures that have no, or little, control over their temperature, because this is a function of the ambient water temperature. These are the poikilothermic animals and to a lesser degree the stenothermic species.
As heat is required for many chemical changes to take place, including those needed for muscle activity, the activity of poikilothermic animals depends greatly on the temperature of the surrounding water. Therefore, these animals mostly flourish where the water is warm, near tropical coral reefs, for example, where there is an abundance of fish species to be seen.
On the other hand, there are relatively few fish and invertebrates to be found in the cold depths of the oceans.
A clear example of what can happen to an ecological system in delicate balance when temperature changes occur is given by starfish and mussels in the tidal waters of Oregon, USA. It was found by Eric Sanford of Oregon State University that a 5ºF (ca 2.8ºC) change is enough to change dramatically the feeding habits of the starfish. This creature feeds mainly on mussels along the US Pacific coast.
He found that a drop of the same magnitude caused the starfish to virtually stop feeding on the mussels, which then allowed a rapid expansion in the mussel population. They can then crowd out algae, barnacles and other organisms. On the other hand, an equivalent increase in temperature caused the starfish to go on a feeding binge, which caused the mussel population to drop drastically. The consequent collapse of the mussel communities then affects the crab, sea cucumbers and worms, which are part of the ecosystem.
His study thus suggests that if a key species in an ecological system is sensitive to temperature, a slight warming or cooling can trigger a cascade of rapid changes that will affect every animal within that system.
Some cold-stenothermic fish, however, may be able to adjust to increasing water temperatures. For example, in the case of the bald rock cod mentioned above, it has been found that when these fish were exposed to long-term changes, they could compensate for those changes.
Dr Frank Seebacher of the University of Sydney has found that they could adjust their cardiovascular system and metabolism to survive in warmer waters. The cod were first acclimatised in 4ºC water for four weeks and then transferred to water with temperatures of up to 10ºC. The fish appeared to live as happily in the warmer water as they did at -1ºC.
However, temperature affects many life history parameters such as fecundity and the development time with the development time from egg to adult being accelerated under increasing temperatures. Increased temperatures can also affect the sex ratios. It has also been found that this accelerating effect of increased temperature has a life-shortening effect corresponding to the rate of reaction increase with increasing temperature. For these creatures, it appears to be a case of ‘Live fast, die young’.
If the changes in temperature occur very slowly, then the species can adapt. The fact that an Antarctic fish, the bald rock cod, has the capacity to compensate for chronic changes in temperature means that we must be careful in our predictions regarding the catastrophic consequences of global warming.
Unfortunately, the current increasing changes in water temperatures are happening too fast for many of the current species to adapt, and they will therefore disappear. Of course, evolution will ensure that species will appear that can survive—and flourish—under the new conditions.
Although the oceanic temperature changes are, as yet, small, they can still have some significant effects. Climatic-related pressures can act directly on aquatic animal life through physiological effects such as changes in food demands, in growth rates, and in abilities to reproduce and survive. It has also been shown that increasing temperatures can be favourable for disease-causing pathogens in corals.
For example, it has been shown that a fungus, Aspergillus sydowii, is a pathogen for the sea fan, Gorgonia ventalina. This bright blue coral, found in Caribbean and Florida Keys, has been dying in some places at a rate of over 20 percent. The sea whips have also been found to be dying of the bacterium Scytonema in the Florida Keys. The optimal temperature for many of these pathogens to flourish is about 32ºC, the temperature at which bleaching is prevalent.
There are also the secondary effects of warming such as predator-prey interactions. These again will have a feedback effect on other plant and animal species, with the bleaching of corals due to increasing water temperatures being a well-known phenomenon.
Coral bleaching
As described in a previous issue, corals are a symbiosis between small polyps and a type of algae called zooxanthellae, which are packed into the cells of the polyp tissue. The algae use the photosynthetic process to produce energy-rich compounds. The corals can utilise some of these products in their own metabolism and, in return, give protection to the zooxanthellae.
Corals and zooxanthellae, like all biological life, live within a preferred range of temperatures. Summer sea temperatures that are 2 – 3ºC above normal can kill corals. At these temperatures, the symbiotic relationship will eventually collapse and, as the corals cannot escape to more suitable environmental conditions, being attached to the reefs, the corals will die.
As it is the algae that give colour to the corals, the loss of them from the coral will cause the corals to become pale or bleached. Many corals appear to be living close to their upper temperature limit, and transient temperature increases of only a few degrees above the usual maximum can kill corals.
However, there is a great variation in susceptibility to changing temperatures between different coral species, so that some corals may survive an increasing oceanic temperature—if not too great. There is also the effect of the increasing solubility of carbon dioxide in the warming surface waters of the oceans, resulting in the reduction of the amount of dissolved calcium carbonate available to reef-building corals.
Apocalypse soon?
Many climatologists today feel like Cassandra of Greek mythology who was thought to be endowed with the power of prophecy but fated never to be believed. So, are we facing another mass extinction event? Not immediately, perhaps, but all the realistic prognoses indicate that there will be a great loss of species by the end of this century. And the great tragedy is that with the bulk of marine species still undiscovered, we will lose species before we even know of them. And it is not just species we will lose, it is whole ecological systems.
In the short-term, it is possible that corals, for example, can adapt to the rising temperatures either by acclimatisation, with changes in their physiology, or by natural selection. However, it may not be the relatively simple effect of an increase in water temperature that is the most important effect. It has been suggested that great changes in the oceanic currents can occur, both horizontally and, especially, vertically.
Such changes could drastically change the environments for all marine species, both the bottom dwellers and those who live in the surface waters. Most species would be quite incapable of coping with such changes and would therefore be wiped out.
More relevant for the diving community, regarding the relatively near future, we may see a total loss of our beautiful coral reefs. So divers, visit the reefs while you can, for many of the more susceptible may not exist for many more years. ■